Batteries fail—as certainly as death and taxes. Rechargeable batteries at least offer the possibility of repeating the cycle, so are in this sense more like recurrent taxes than death. But alas, the story cannot repeat indefinitely. One cheerful thought after the other, yes? But wait, there’s more… Add to their inevitable demise an overall lackluster performance in battery storage technology, and we have ourselves the makings of a blog post on the failure of batteries to live up to their promises.
To set the stage, the specific energy of gasoline—measured in kWh per kg, for instance—is about 400 times higher than that of a lead-acid battery, and about 200 times better than the Lithium-ion battery in the Chevrolet Volt. We should not expect batteries to rival the energy density delivered by our beloved fossil fuels—ever.
A recent article in APS News reported on an emerging view that batteries are failing to live up to our dreams in the electric car realm:
Despite their many potential advantages, all-electric vehicles will not replace the standard American family car in the foreseeable future. This was the perhaps reluctant consensus at a recent symposium focused on battery research.
I was somewhat stunned to see this article. I am accustomed to seeing articles emphasizing the possible—albeit often improbable, in my mind. Also appearing in the article is a quote from Paul Alivisatos, an accomplished physicist, summarizing the need for further research:
“It remains true today, as in the past, that we need a fundamental understanding of the physics of how energy-conversion processes take place, at a much deeper level, in order to achieve a truly sustainable energy future.”
Rephrasing: the physics we currently understand is not sufficient to deliver the kind of battery we need to make the future work without fossil fuels. Red flags go up for me when it is our understanding of physics rather than practical engineering challenges standing in the way—as serious as the latter can be. Physics limitations instantly present a much taller order to overcome.
Anecdotes
I’m sure everyone has tales of how batteries have let them down—ranging from the merely annoying to life-threatening situations. I find that I am more often disappointed than pleasantly surprised when it comes to batteries. Here are some examples:
- I frequently go for months without driving my truck. The battery is often dead when I try to start it. Lead-acid batteries only get worse if left in a discharged state, so it’s a runaway process. Fortunately, I live on a hill and can often roll-start my way back onto the road.
- The rechargeable NiMh batteries I use for small electronics devices are rated for 1000 charge cycles. I’ll bet I only get about 15–20 cycles before noticing a serious degradation in performance.
- The first set of lead-acid batteries I used with my home-built solar photovoltaic system only lasted two years before showing substantially reduced capacity. A newer set is still in good shape after 2.5 years, but the drop in performance can be pretty fast, I have found.
- Lead-acid batteries for cars tend to last 5–6 years, often failing with little warning, in many cases resulting in being stranded.
- New laptop batteries seldom fail to delight their owners in how much longer the charge lasts compared to the previous generation batteries. But give it a few years and it is not uncommon to be operating at half the original capacity.
- Batteries left in a device for a long time can develop corrosive crud around the terminals, often in hard-to-clean places.
A counter-example is the occasional amazement I experience when alkaline batteries in a device that has not been utilized in years crackle to life after all that time—if the batteries haven’t gooped themselves up, that is.
Energy-Power Tradeoff
The chief measure of a battery, in my mind, is how much energy it can store. But it makes sense to adjust this concept to the size or mass of a battery. Obviously, a more massive and voluminous battery can pack in more energy. So for a given mass (we’ll take a kilogram), we want to know how much energy a battery can store, called specific energy.
At low power demand (sipping rather than gulping), lead-acid batteries tend to hold about 30–40 Wh per kilogram (one Watt-hour is equivalent to 3600 J, or 0.001 kWh of energy). Ni-MH batteries score 45–60 Wh/kg, and Lithium-ion gets about 120–180 Wh/kg. Part of the reason for Li-ion’s better performance is that lithium itself is lightweight; by volume lead-acid has about 40% the capacity of Li-ion. Gasoline, at 36.6 kWh/gal, has a specific energy of 13,800 Wh/kg. Off the charts!
As power demand increases, the battery flags, and will not offer as much total energy. Obviously, the battery discharges faster under heavier power demand, but the effect is exacerbated by less actual energy available. This is best shown on a Ragone plot, in which specific energy is plotted against specific power.

Characteristics of various battery chemistries, plus a few other technologies thrown in. Diagonal lines indicate time to discharge. The various electric vehicle goals indicate maximum power desired, but discharge times only apply if the maximum power is sustained until complete discharge, which is not the way we drive (most of us!). Plot courtesy of V. Srinivasan.
Note that the Internal Combustion Engine (ICE) exceeds both specific energy and power goals for vehicles (the mass must include engine weight, rather than the fuel by itself). Fuel cells provide decent specific energy, but typically insufficient power (per kilogram). Capacitors, including super-capacitors, discharge super-fast with lots of power, but have very low specific energy.
As useful as this plot is, it does not convey the whole story. While it looks like Li-ion meets the the goal for plug-in hybrid electric vehicles, this does not necessarily remain true if demanding 5,000 deep charge cycles, a ten-year lifetime, a moderately inexpensive product, etc.
Spider Diagrams
The U.S. Department of Energy teamed up with the automotive and battery industries to define benchmark performance targets for batteries that would result in electric vehicles being competitive with ICE vehicles on a mass-produced basis. The resulting coalition was called USABC/FreedomCAR, and their various target requirements are available here, with a useful summary presentation also available. Below is a subset of the target parameters pulled from these sources, and I have also thrown in the Chevrolet Volt for a side-by-side comparison to current capabilities.
Characteristic | Hybrid Goal | Plug-in Hybrid Goal | Chevy Volt (PHEV) | Electric Vehicle Goal |
Range on Battery (mi/km) | 1.0/1.6 | 40/65 | 38/61 | 300/580 (?) |
Available Energy (kWh) | 0.3 | 11.6 | 10.5 | 40 |
Specific Energy (kWh/kg) | 0.007 | 0.097 | 0.053 | 0.2 |
Energy Density (kWh/L) | 0.009 | 0.14 | 0.105 | 0.3 |
Specific Discharge Power (kW/kg) | 0.625 | 0.32 | 0.56 | 0.4 |
Power density (kW/L) | 0.78 | 0.47 | 1.1 | 0.4 |
Cycle Life (cycles) | 3,000 | 5,000 (deep) | 2,000 | 1,000 (deep) |
Calendar Life (years) | 15 | 10 | 8 | 10 |
Production Price (USD) | $500 | $3,400 | $8,000? | $4,000 |
Operating Temp. Range (°C) | −30 to 52 | −30 to 52 | −25 to 50 | −40 to 85 |
The 300 mile (580 km) range for the pure electric vehicle (EV) comes from the presentation rather than the official USABC source, and does not look right to me based on the 40 kWh battery size. Electric cars typically need 30 kWh of storage for each 100 miles of driving (about what the Volt, Leaf, and Tesla achieve, based almost entirely on air resistance—not battery technology). So I would expect the 40 kWh battery pack associated with the EV goal to deliver half as much range as what’s in the table.
Some of the figures for the Volt deserve explanation, since many cannot be directly looked up, and require inference and calculation. Firstly, the 2013 model battery pack has a capacity of 16 kWh, but only 10.5 kWh are made available so-as to avoid potentially damaging deep discharges. Meanwhile, I have no choice but to use the entire battery pack mass and volume (197 kg; 100 L) in conjunction with the partial 10.5 kWh charge in calculating energy densities, because available energy density is what’s important. For lifetime and cycle computations, I use the 100,000 mile, 8-year guarantee on the battery, together with the estimated 37 miles per gallon (MPG) on gas alone and 98 MPG for combined gas/electric. This implies an expectation that about 62,000 of the 100,000 miles will be driven under battery power. If recharges typically happen after 30 of the 38 miles are spent (corresponding to 80% of available capacity), this translates to about 2,000 deep cycles. Perhaps this is pessimistic in the sense that most guarantees correspond to a minimum expected performance. But offsetting this is the fact that the USABC targets are specified for end-of-life performance, whereas I use the beginning-of-life numbers for the Volt. General Motors estimates a 10–30% degradation at the end of 8 years (100,000 miles).
A comparison between actual performance and target performance can be cleverly displayed graphically in a “spider chart,” as illustrated below for the plug-in hybrid performance as of May 2011 (I first saw such diagrams in a presentation by Venkat Srinivasan, in 2008).
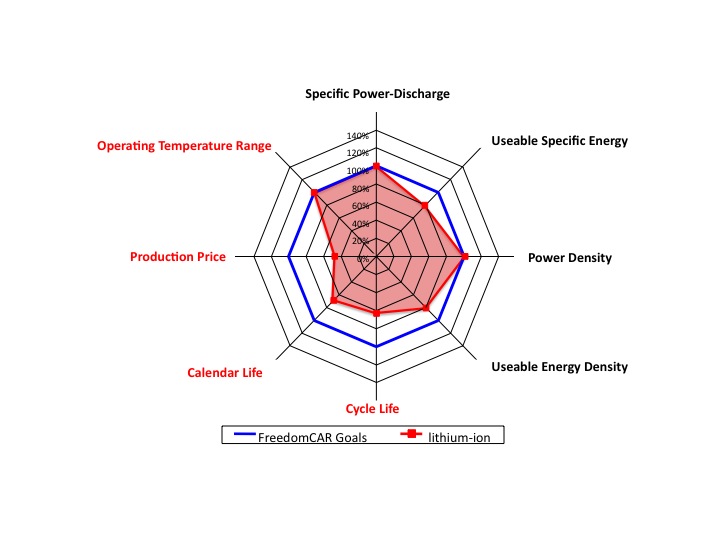
Figure from an LBL news article showing current battery performance relative to PHEV targets (blue line). The story is dominated by underperformance on many criteria.
We can make our own spider diagram for the Volt, based on the numbers in the table. Please excuse the sub-optimal placement of labels, etc.
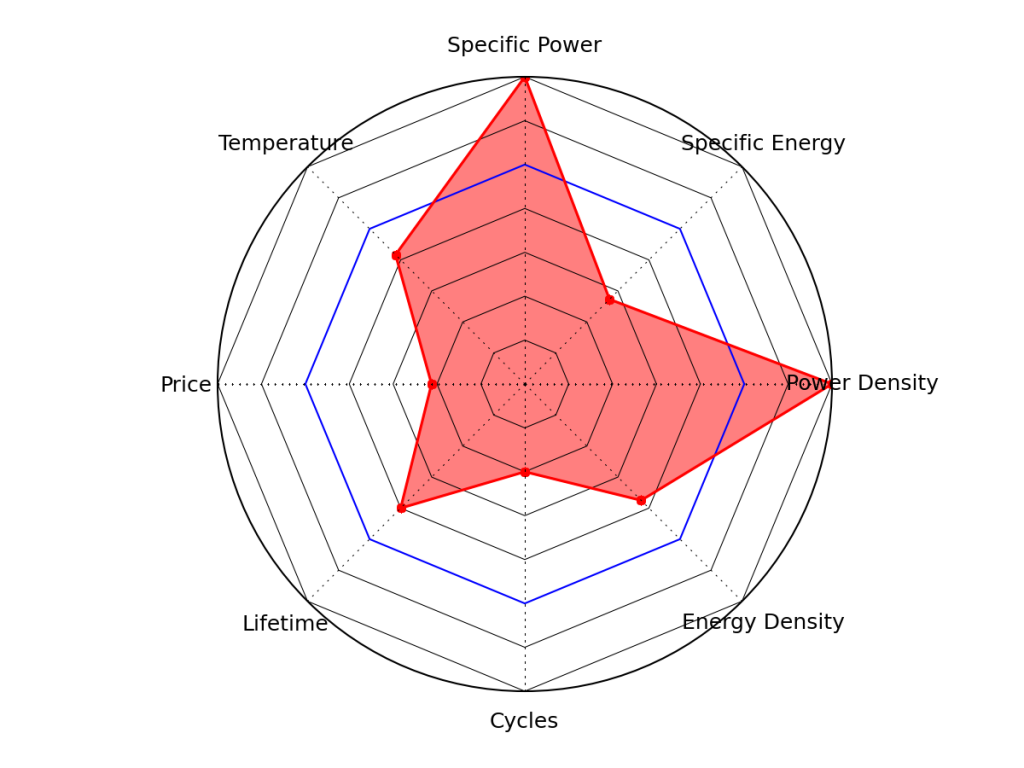
Spider chart for the Volt in relation to the USABC/FreedomCAR targets, using my estimates for several parameters, as described in the text. Power performance exceeds the values plotted, so the points are simply placed on the peripheral rim as an indication of sufficiency.
Besides looking like some sort of cool fighter jet in a dive, the diagram highlights performance deficits on several fronts. It is not terribly hard to get lots of current out of a battery, translating to more-than-adequate power performance. But all other measures fall short of the goals by varying degrees. The APS article intones that we should not hold our collective breaths to see a march of progress in lithium-ion technology at a level that would satisfy this (still hungry) spider. In practice, improving one aspect of performance tends to decrease another somewhere else (see the piece by Srinivasan for more on this principle). So it’s not a simple matter of advancing on all fronts independently and incrementally.
Full Cost of Electric Drive
Let’s say you pay $0.10 per kWh for electricity delivered to your home. Charging the Volt battery with 10.5 kWh at 90% efficiency to replace the drain from 38 miles of driving will cost $1.17. If using gasoline alone, the same car uses about a gallon of gas to go the same distance. Let’s put the cost of that gallon at $4.00. Electric looks pretty good, at these rates!
Now figure in the estimated price of the Volt battery at $8,000 (a disputed number, but GM has not revealed the actual cost). If we get 62,000 miles of electric drive out of the battery, we will spend $1950 on electricity for charging, plus $8000 for the battery. That’s $9,950. The same distance on gasoline would cost $6500. Not an order-of-magnitude difference, but still gasoline currently wins.
If the price of gasoline goes up (it will; but so will electricity), and the cost of the battery goes down (it should), the two may cross. But there are other added costs to the Volt (or hybrids in general) besides just the battery. After all, hybrids can’t jettison the ICE, and require an electric drive train to boot. Even the fact that the space occupied by the battery forces bucket seats in the back of the Volt is a “cost” that must be paid.
Beyond Cars
Batteries are, of course, useful for purposes other than transportation. While transportation hardship may be the most pressing problem in the decades following peak petroleum production, solar and wind resources cannot scale to be very large without a viable storage solution.
I worked out in an earlier post how large a lead-acid battery would have to be to support the entire U.S. energy demand in the presence of solar/wind intermittency. It turned out that our estimates for recoverable lead in the world do not satisfy the need. Lithium and Nickel are even more constrained. It is possible that some other approach like sodium-sulfer or zinc-air can step in. But these are already relatively well-known options and have not blazed a wide path into storage over the past few decades.
Sigh
Don’t get me wrong: even though I dwell on the shortcomings of batteries in this post, I still hold a net positive view. When it’s dark at my house, my refrigerator, television, computers, and internet goodies are all powered by stored sunlight in lead-acid batteries. My laptop battery gets me through many a bus ride and an occasional airplane ride. Batteries really do work, and provide value. Moreover, electric cars are more than a notion or fantasy: they are actually on the road getting people where they want to go. Despite their lackluster performance next to fossil fuel storage, batteries still beat the pants off of mechanical or gravitational storage.
And even though I might appear to be picking on the Chevy Volt by highlighting its deficiencies, I actually rather like the design point (electric vs. gasoline range hits the sweet spot, in my view). In fact, I was half way to buying one. By half way, I mean that if the price were cut in half, I would surely have one now.
The real point is that batteries fall pathetically short of our customary fossil fuel energy storage medium. When we wake up to a declining global availability of petroleum, we won’t just switch over to electric cars. We may not be able to collectively afford such a transition, given the huge up-front costs in both money and energy. Where will the prosperity come from? If oil shortages drive recession in the usual fashion, expensive options may be off the table.
Addendum
The same author of the APS article referenced above wrote an extended version, worth a look.
Hits: 6676
The point about fundamental physical limitations is the real killer here – you are never going to store as much energy as there is in a chemical bond in a battery, or in gravitational and mechanical devices. That’s how the physics works.
The inevitable conclusions then is that we need to look at what the possibilities are for storing energy in chemical bonds. Hydrogen is not practical and there already are large conversion losses with it, so I would expect there would be even larger losses with more complex compounds, but I am not a chemist and don’t know the details – I would love to see someone explaining what the possibilities and limitations are, but haven’t seen that done anywhere. The problem, of course, is that given the conversion losses, one has to scale up the renewable energy harvesting capacity accordingly, and we already have a huge problem with scale there, so this is only going to make things worse. Still, in the impossible scenario, in which we are willing to scale human civilization down to within what is realistically supportable by such means, this would be a better solution than batteries.
A clarification on the initial statement: batteries do store energy in chemical bonds. Gravitational and mechanical storage tends to be far weaker than batteries, even. See the post on home energy storage to see by what margin gasoline beats batteries beats compressed air beats flywheels, and all beat gravitational by a large margin. I added a sentence in the last section to this effect.
I know how batteries work, I didn’t use the words “chemical bonds” in that sense. I use them in the spirit of what others have posted below too – that hydrocarbons are the most convenient energy storage we have and as batteries will never come anywhere close to matching that, we should see what the options are for storing energy in the form of hydrocarbons (or something else with similar characteristics if it exists). As I said above, I have not seen much discussion on the subject and I can only find a few scattered articles on the subject here and there, i.e.like this one:
http://www.sciencedirect.com/science/article/pii/S1364032110001942
Of course, the usual constraints of practical scalability and efficiency apply, but it would be great if we knew what exactly they are based on a sufficiently large body of research on the subject.
Fraunhofer Institute is researching process to turn solar power to gas to use already built storage capacity.
http://www.popsci.com/science/article/2010-05/carbon-neutral-natural-gas-made-wind-and-solar-could-power-existing-infrastructures
http://www.brighthub.com/environment/renewable-energy/articles/78303.aspx
I would say this is the best option we currently have to economically store solar/wind power.
GM:
I am with you. Rather than techno-grandiosity (JHK) solutions to our energy problems (i.e.. better batteries), a planned depopulation to a billion of less humans on the plant is what we should be addressing. That, of course, won’t happen, but Mother will take care of it.
Oh but it will….! I won’t be around, unfortunately, but I would expect world population to collapse to a billion by 2100, simply from lack of food caused by Peak Oil and Peak Phosphorus.
http://damnthematrix.wordpress.com/2012/04/11/so-much-for-debunking-the-club-of-rome/
Good article, battery technology is something I would really love to know more about. Is there any future battery research you have heard of that shows big promise right now?
The APS article mentions lithium-air and lithium-sulfer as technologies of interest, but points out that the practical difficulties are enormous. So my take is: don’t hold your breath.
Did you heard about Envia systems battery ? They GM backed start up and had successfully tested battery with 400 (Wh/kg) density and claiming that the cost is a half as much as today batteries http://enviasystems.com/announcement/
“If the price of gasoline goes up (it will; but so will electricity)”
The parenthesis seems gratuitous, here. We expect peak oil in its various forms to drive up the price of gasoline. But, especially in the US, electricity doesn’t have much to do with oil prices, and “peak coal” or “peak methane” needn’t happen at the same time — and have more ready substitutes.
There’s also the environmental subsidy IC cars currently enjoy, which could be accounted for (and is somewhat, inefficiently, by EV subsidies.)
We also can already set an upper limit on the peak price of electricity: today’s cost for photovoltaics. And, while PV is more expensive than coal (ignoring the price of coal’s pollution), it’s cheaper than many of today’s standby peak power plants.
That still leaves baseload power, but we should be able to set some limits on that, as well. What would the cost electricity be with today’s technology from a gigawatt-sized PV plant with iron Edison-style batteries providing overnight baseload power? That’s the upper limit on baseload power — which, in an inversion of today’s market, will likely be more expensive than midday peak power.
If I had to hazard a guess, the price would be no more than triple today’s rates. And that’s a price that should get cheaper with time, not more expensive.
Cheers,
b&
You make two assumptions. The first is that production of coal and solar panels is entirely independent of oil. It might well be possible to make them independent but I doubt they are at present. Oil increases ->transportation, plastic, food, etc all increase relative to the cost of human labor.
The second is that these things are not used as a substitute for oil. Coal can be turned into liquid fuel. Electric cars are obviously substituting other materials and electricity for oil. Both of these uses would drive up the price of electricity.
I read an article a while ago (sorry can’t find link) that stated battery technology has at best a 2x improvement possible based on underlying physics. Kind of similar to your earlier efficiency improvement analysis. There will be no miracles.
The future will be gas scooters and electric golf carts followed shortly by walking and horses for the rich.
If there’s only a 2* potential improvement in batteries available then they’ll never be a direct replacement for gas/petrol/diesel. But that doesn’t mean they are useless. Perhaps it means we need to adjust our expectations and approach. Perhaps an electric bicycle makes more sense for a short trip to the shops for milk than an SUV.
Meanwhile, I’m deeply suspicious of the claimed specs given by car manufacturers. They seem to be more optimistic than A123, and specs from A123 are probably more optimistic than actual reality.
I agree that the best application for battery power would be small vehicles and shorter trips… but let’s not drop all the way down to bicycles!
I think it is insane that the typical method for getting around is a 4000+lb vehicle hauling a payload of one human. If we focused on enclosed vehicles with a modest size (500lb), speed (45mph max), and range (40 miles), electric vehicles would be very viable with current technology.
But before people will “want” to use such a thing we’d need to reconfigure our infrastructure so that they are safe and easy to use… ie favor them and restrict the use of larger vehicles in urban areas. A typical family might have a couple of these small cars for commuting to work or short trips, in addition to a larger vehicle for hauling loads and long trips.
A bonus would be reduced congestion in addition to much lower energy consumption and pollution.
Totally agree. I’m planning on converting a one ton (load capacity) 4 cylinder pickup to electric drive, just for the purpose of carrying heavy loads short distances (under 25 miles). That I will be able to recharge the batteries using rewable energy is a plus….
http://damnthematrix.wordpress.com/2012/05/18/ive-bought-a-car/
Spider plots aren’t very good at showing what’s happening (circular displays in general aren’t, but people think they look cool). Better to straighten them out into a parallel coordinates plot. A bonus is that you can now show many different profiles on one plot.
But then you loose the cool jet fighter! 🙂
Tom,
It seems to me that the ultimate goal isn’t to store electricity, but to store energy in a compact and portable form. And we already know that hydrocarbon fuels are a fantastic compact and portable form of energy storage. We can also generate electricity quite readily from hydrocarbons, too.
How do the various methods of creating (not mining) hydrocarbon fuels compare to today’s conventional electric batteries? I’m thinking of both Fischer-Tropsch syngas synthesis and biofuels…but only because those’re the only two such methods I’m aware of.
I’m not sure where one would start on figuring out how to make the comparison, which is why I ask. Perhaps the cost per 3,000 gallons of gasoline-equivalent fuel, since you’re already comparing the lifetime costs of batteries and conventional gasoline?
Cheers,
b&
Another nice article! Keep up the good work.
One additional thought on your cost comparison – Your compared the lifetime cost of running a Volt on its electrical system to its gasoline engine and found the gas cost slightly less (when battery depreciation is included). The ‘average’ US vehicle gets about 1/2 the mpg of the Volt in its gas mode, however, making the Volt operational cost cheaper than the ‘average’ vehicle.
Also, an additional factor one might consider is the amount of carbon pumped in the air. For an electric vehicle (or a plug-in like the Volt), this depends on the source of electricity used to charge the battery. It turns out (see http://www.dhiggins.org for details) that a Volt charged from a natural gas-powered generation plant produced less CO2 than a gasoline powered vehicle when the gasoline mileage is less than roughly 45 mpg. So a gasoline powered vehicle may produce less green-house gases if it has a high enough mpg.
Now I did buy a Volt (despite its high price), but I also have a 20 panel, grid tied PV solar system that produces enough energy over a year to power both my home electrical use and charge the Volt. An electric vehicle powered by solar cells produces about an order-of-magnitude less CO2 that one powered from the grid! So an electric vehicle power by PVs rather than the grid is a winner in terms of CO2 emission. [And the Volt is a heck of a lot of fun to drive!]
I’m a Volt owner. Its true that the price scares people away, but owning over an average period (6 years) is cheaper than owning the average car ($30,000 price), in fact it beats it even without the tax rebate. I’m not sure where you came up with 62% electric miles, my average after one year of ownership is 88% electric miles. Your estimate of the replacement cost of the battery is wrong, its listed in the GM parts catalog for $2,995 right now. Is that the _real_ cost? Probably not. But in 8 years its probably about right based on decreasing costs of production. Also I charge my car overnight at a discount, $0.055 / kWh. And the charging efficiency of the Volt is 84%, not 90%.
Use those figures and the Volt costs $3,000 + $1,590 electricity = $4,590 for 88k miles. Meanwhile, 88k miles at 37 MPG and $4/gal = $9,500. Pretty much the opposite of your conclusion.
Don’t forget, at 8 years the Volt battery is NOT dead, its warrantied to have 70% capacity, and GM engineers recently said they were TOO conservative with the 2011/2012 models (mine is 2012), so its likely to be better than that. Also the Volt already has above average resale, which is actually a dominant factor in any analysis of car ownership.
The 62,000 miles came from the sticker numbers for the Volt: 98 MPG combined and 37 MPG on gasoline. I just did some math. Individual practices will certainly vary. But this presumably matches some expected overall driving pattern, on which the 100,000 mile warranty is also likely based. Great that you get a discounted rate on charging!
The 100,000 mile warranty is not based on driving patterns, the car manages the state of charge and temperature of the battery at all times, they’re confident the driver can’t do anything to screw that up. Like I said, they now think they were too conservative in only allowing 10.4 kWh to be used out of 16. The 98 MPGe rating is such a contrived number (check out its origin) its not even worth mentioning.
Average across all age groups is 13,500 miles per year. Median Volt driver is at 80% EV miles. That’s 10,800 EV miles per year (~30 per day) and 86,400 EV miles in 8 years. The costs have already “crossed” as you put it, I think a closer analysis may cause you to update this article, or at least show a best case / worst case comparison.
Your anecdotal evidence is not applicable to the Volt battery. The Volt keeps the battery in an ideal temperature range (as much as possible), including starting the engine automatically to warm it below 26 F. The battery is never charged to its maximum potential, and never discharged below ~30%. Cell phone and laptop batteries die because they’re constantly being charged to their maximum potential and discharged fully – something that will never happen in a Volt. GM’s target was 10 years and I have no reason to believe that’s unrealistic.
Normally I’m impressed by your deep knowledge about the subjects of your articles, but I have to say your analysis of the Volt is wanting. Stating the unsubstantiated $8,000 battery price that GM never confirmed and I specifically pointed out was false in the comments of another article?
It’s true that we don’t know how much the Volt battery costs, and that $8,000 is perhaps merely rumor. But it’s not out of line with typical costs of $500 per kWh ($470/kWh for Leaf battery, with ambitions toward $370/kWh). Multiply $500 by 16 kWh and we have $8,000. So no red flags for me. In any case, it’s mildly disturbing that we are not allowed to know the true cost.
I’ll at least add a comment that the $8,000 cost is disputed (in addition to the question mark already in the table).
Your math was based on end user replacement cost at end of life, not manufacturer cost today. Its doesn’t matter if it costs GM $8,000 to build it right now, they’ll sell it to me for $2,995, making the $8,000 not only disputed but demonstrably false.
http://parts.nalleygmc.com/products/Chevrolet/Volt-LTZ-4-DOOR-HATCHBACK/BATTERY-Drive-Motor-Battery/2949468/20979876.html
It will be worth watching what the price goes to in ten years when there may be actual demand for replacement. We all might hope that the $3,000 holds, but is there any guarantee? Will GM be supporting the Volt in 10 years? Does the fate of EV1 have any bearing? I put more stock in actual costs now than projections or hopes for the future. If GM will sell a battery pack right now for significantly less than the actual cost, this reflects a mixture of long-term planning and marketing (don’t want to scare potential customers away). But GM won’t be interested in losing money indefinitely, if things break this way.
I do hope you’re right that the future cost will drop to the $3,000 mark, but meanwhile I am interested in doing a real analysis of what it actually takes today.
“I do hope you’re right that the future cost will drop to the $3,000 mark, but meanwhile I am interested in doing a real analysis of what it actually takes today.”
No, you’re not, I proved you wrong, in fact I found another dealer selling it for $2500:
http://www.gmpartseast.com/parts/gm-20979876_battery.html
No, the death of the EV1 has no bearing. You don’t know much about auto production do you? EV1’s were leased, which is why GM could take them back an crush them. When you _sell_ a car in the US you are obligated under Federal law to provide replacement parts while the vehicle is under warranty. So, no, the EV1 is not an example of what may happen to the Volt. You’ve obviously branched off into a topic you’re not equipped to analyze.
Steve,
I think what Tom is saying is that it is easy to list a replacement battery at below cost when there is little to no demand for replacement batteries (demand for end-of-life replacement won’t really kick in for years yet).
That GM might be willing to take a hit on the handfull of replacement batteries they currently sell for some marketing advantage is not reason to believe that these batteries will always be sold at this price point.
In the absence of actual cost information from GM, I’d say it fair to assume the dealership price is more “smoke and mirrors” than an indication of what these batteries actually cost to manufacture.
A big question would be whether they’d sell the battery to somebody who doesn’t own a Volt…which I suspect they wouldn’t. Most likely, that $3,000 figure only applies if you give them your old dead battery — much like the standard “core” fee for transmissions, engines, alternators, and other parts that get rebuilt.
$3,000 for a Volt battery would be pretty awesome for a do-it-yourself electric vehicle conversion, as I understand the market. If they really will sell the battery to anybody for that price, you can probably expect a pretty significant wave of such conversions to hit the road in the immediate future.
With a single Volt battery costing $3,000, you should be able to get something that outperforms the Leaf for under $15,000, including the cost of the donor vehicle and excluding labor. That’s a deal I’d personally find most tempting, myself.
Cheers,
b&
The problem is that he’s conflating end user energy costs and manufacturing costs. It very well may cost $8,000 to produce today, so what? If they’re willing to sell it for $2500 they clearly believe that by the time there is a demand, the manufacturing costs will have dropped to that level. His math assumes no decrease in battery manufacturing costs in 8 years (zero chance, its dropped in half in 3 years) and no increase in the price of gas in 8 years (near zero chance).
Charging efficiency of the Volt is apparently a function of the charger. I charge mine with a standard 120 volt socket and I measure about 80%. I have seen the efficiency rated at 90% several places if one used a 240V charger.
90% efficiency while charging is too generous on the Volt. My Kill-a-watt shows the Volt pulls 1440 W and takes 10 hours to charge (10.5 hours with the 2013). That is with the standard 120. I’ve read the 240 charger is a little over 10% more efficient.
I think the price for the Volt is fair, just as I think the price for the Tesla Roadster is fair.
I just have no interest in paying anywhere near that much money for a car.
I would be interested in paying $20,000 or less for a plugin hybrid with performance similar to that of the Volt, even if that meant getting a compact no-frills econobox (but built to last a quarter million miles or more and with A/C…I am in Arizona). Think of a VW Golf or a Honda Civic or a Toyota Corolla, but with a plugin hybrid motor. Tom, I hope that’s the sentiment you were trying to express with the bit I quoted.
Sadly, I fear we’re likely at least a few years, if not more, away from such a vehicle.
Cheers,
b&
Iron-air batteries audition for grid storage role referring to A High-Performance Rechargeable Iron Electrode for Large-Scale Battery-Based Energy Storage J. Electrochem. Soc. (2012) 159(8) A1209-14 doi: 10.1149/2.034208jes
may interest.
Batteries are, and will remain, a sticking point, but I think it is possible to maintain a mobile society based primarily on electric power (ignoring air travel for now). Here’s how I would do it:
1. Rather than designing big, heavy electric vehicles (and complaining when batteries fail to perform), design small, sleek ones with the efficiency advantages of motorcycles but with familiar aspects (enclosure, steering wheel and controls, safety frame, seat belts) of cars. I’m personally a fan of the Arcimoto (http://www.arcimoto.com/). Aim for a range of around 100 miles, with fast-charge or battery-swap technology so that longer drives are possible.
2. Provide biodiesel-powered pickups and vans for rent, which people can use on those rare occasions when they really need cargo or passenger capacity.
3. Electrify rail transport networks and add induction or overhead wires (http://dvice.com/archives/2012/05/overhead-highwa.php) to freeways, thus making possible electric buses, trains, and long-haul trucks.
4. Provide an affordable pool of electric vehicles (of the same type described in #1) for rent near transit stations, making it easy to take a trip to a different city by electric bus/train and still retain the personal mobility so treasured by Americans.
Does anyone else find it odd that the only widely-accepted types of vehicles are 20-pound human-powered bicycles and 3500-pound gas-powered cages of steel? It is possible to go reasonably fast (50-60 mph) and reasonably far (~100 miles) with existing battery technology if we accept smaller, lighter vehicles designed with one goal in mind: getting people safely from point A to point B using the least amount of energy.
Well said, but electrification of even bigger vehicles is the way to go… meaning public transit vehicles. We can’t ignore the need for private transport options, but if we want o move hoards of people at low energy cost we have to go beyond that.
Looking at the Arcimoto, I can only call it a first step at convincing people they don’t need quite such giant wheelchairs; it’s still much larger than a normal small motorcycle.
If you include China in your popularity poll, you’ll find a bunch of people using either electric-assisted bicycles or plain electric scooters, so there is a 3rd choice that is somewhat heavier and faster than a bicycle, though also less safe than either bicycle or car. Note that in many places the theoretical top speed of a vehicle is irrelevant; if the usual use (the one that consumes most time and energy) is commuting in a traffic jam, that is all the speed that is needed. See here: http://www.flickr.com/photos/jurvetson/4503260722/#comment72157630081967672
As far as “safety” goes, that is purely a matter of marketing (i.e., keeping consumers uninformed). Crash risk is dwarfed by the risk of using a car (any car, large or small) to the exclusion of sufficient exercise. http://dr2chase.wordpress.com/2010/07/16/us-non-cycling-excuses-safety/
The advantage of the smaller vehicle is that batteries are much more easily swapped, and can be charged much more quickly without requiring an unusually large (for a household) power feed.
The price per kilowatt hour seems like the most deficient aspect of current batteries. It’s also the hardest to track, rather like trying to find GM’s internal manufacturing cost for a transmission or other major component. Lithium ion batteries would already be good enough for passenger cars on technical merits if the price came down enough. (“Don’t be a Specific Energy Snob.”) But I understand that “current” prices are around $500-$650 per kilowatt hour, in automotive capacities and configuration.
I don’t know what the expensive parts of turning (relatively) cheap minerals into lithium ion batteries are. Lately battery-grade lithium hydroxide is selling around $6 per kilogram, and that should be by far the most expensive mineral component of (e.g.) a lithium iron phosphate battery from A123 Systems. But the manufactured battery costs something like 20-30 times that of the bulk price for the purified materials that go into it. If PV manufacturing had a similar cost ratio between purified materials and full modules, silicon-based modules would still cost more than $5 per watt.
Are we just waiting for manufacturing experience, scale, and competition to bring prices down, like silicon PV prices fell over the last 5 years without any revolutions in physics or chemistry? Are there greater fundamental challenges in making batteries like today’s but cheaper?
I’ve been skeptical of batteries powering cars for a while now, and this post mostly reinforces what I already thought. It seems liquid fuels will always be superior.
By the by, will you be doing a post on airborne wind energy? I’d love to get your take on it, given that it’s a rapidly emerging energy field.
You don’t need to be skeptical of batteries powering cars, that’s how I get to work every day 😉 Liquid fuels will always be more energy dense, however future availability (Peak Oil) will make them somewhat less than superior.
It is clear that the specific energy of gasoline is much greater than any existing(or envisioned) battery. However, we should remember that the efficiency of the internal combustion engine in using gasoline energy is only about 30% and that this is limited by fundamental thermodynamics. Electric motors, on the other hand, have efficiencies of more than 90%. Electric vehicles thus have an advantage in the overall efficiency of converting stored energy to motive power.
I remain cautiously optimistic.
Electric motors are close to 4x as efficient, are based on an already existing infrastructure that delivers at the speed of light and which can be powered by solar and wind via power lines potentially spanning the globe thus reducing massive storage requirements significantly. I know we need fossil fuels and nuclear as back up but we can clean them up (somewhat) as well. Speaking of nuclear, ehh, we might still need batteries depending on how expensive methanol production via heat process would be (?)
I believe we have the ability to mine enough lithium for the LFP (or LiFePO4) battery. Extracting 1/10th of 1% of the ocean’s content would provide some 15 – 20 billion Tesla car type batteries, you know, the ones we want. However, I recommend the LFP even though not quite the specific energy. Why? Because it does not have the thermal issues and has at least twice the cycling life.
I have searched “tests” on youtube… the li-ion always “acts up” whilst the LFP remains “cool” (even when severely overvolted complete with nails hammered through and even torn apart)! I believe, it’s something to do with the iron phosphate replacing the manganese. Does it really matter if a car is a few percent larger due to having to have a large (but lighter and safer) battery pack? It still beats sourcing out the work to the backs of biological forms!
If solar is “almost good enough” then so is the LiFePO4 battery (at least for mobility).
Oh,
LiFePO4 aside, once the “prevailing” tech is agreed upon, in a peak oil scenario, wouldn’t competing companies “do it all”, that is own the mining lease, own the equipment, own the robotics (to make all parts) own the trucks and even own the stations where batteries are swapped while customers are buying a soda?
Tom – your doubts of the future mass sales of battery powered vehicles on grounds of both physics and economics make sense to me. There is also the ecological replicability issue in terms of the hit of the materials extraction-transport-processing needed to build and maintain a global vehicle fleet of perhaps 2.5 billion in the coming decades, from the present of around a billion. If a technology doesn’t work for the world, then it surely won’t work for America.
The ‘headwinds’ faced by US prosperity have yet to be widely appreciated, but it’s already plain that there is an inability to maintain infrastructure, to educate sufficient young people for international competitiveness, to conserve scarce vital resources (see Vegas v Ogallala Aquifer) and so forth. The impacts of PO and AGW are not unprecedented threats to an empire in decline, but the fact that they’re both global in reach and are also accelerating raises their potency in terms of eroding prosperity.
Lousy elite response seems to be a characteristic of fading empires and the US appears no exception. For example, Cheyney’s climate policy of a ‘brinkmanship of inaction’ with China, whose adoption Obama signalled within weeks of taking office, faces that superpower rival for global economic dominance with the looming prospect of climatic destabilization. But the policy is based on the flawed assumptions that China will be harder hit by climate impacts than the US (despite the common exposure to the Jet Stream’s disruption) and the US will be better able to endure the losses (despite China being the rising economy, dilapidated US infrastructure, disparity in the political impact of casualty figures, etc).
From this perspective, the double failure to rebuild New Orleans, on higher ground, was perhaps the high profile tipping point into a trajectory of decline. So maybe identifying future private transport demand needs to start from that reality ?
A very large but relatively poor cohort of drivers can be seen in China, where diverse forms of electric bike are already in use by over 100 million people, who find them much better than the alternative, of walking. With a rising cohort of young Americans disdaining or excluded from car ownership, and over 30 cities launching pay-per-use pedal-bike rental schemes so far, effective electric bikes look like a strongly rising prospect in the coming years, gaining social chic and meeting the need for affordable transport – at the expense of the market for EV & HEV.
At issue is the optimum form of energy store driving the power train, with energy density being a still higher priority on the AUW of a bike than even a sleek car. We’re agreed that battery techs aren’t going to outrun hydrocarbons on this measure, so how about a post looking at the viable production scale of that most sustainable of biofuels – namely coproduct methanol (off charcoal production for carbon sequestration and soil enhancement) drawing feedstock from widespread afforestation in the form of coppice forestry ? The fact of around 8.6Gts of dead wood so far (due to the bark beetles’ boom) is now waiting to rot or burn, or be used as above for feedstock, is a further consideration.
As you’ll know, the Nobel laureate George Olah wrote a book proposing “The Methanol Economy” a few years back, basing the fuel’s mass production on air-capture of carbon at huge new hydro-power stations built for hydrogen supply on very remote prime topography sites. Your thoughts on the global potential and viability of his proposals would be much appreciated.
A third potentially major source is the possibility of mooring wave energy conversion vessels beneath the prevailing 12 metre waves of the ‘roaring forties’ far south of Australia, and piping or tankering the products ashore. While this is plainly an extreme environment for production, it offers extremely high energy gain per unit of infrastructure, which is the basic metric of the energy systems we require in production just as much as in transport usage.
With regard to the use of methanol [CH3OH] its exceptional clean burning properties make it ideal for mass urban usage, while its speed of combustion combined with low fire-risk further raise its utility as a bike fuel. Some will of course assume it should be refined to petrol to roughly double its energy density, but given its advantages and its massive improvement over the best batteries, I doubt that it’s worth the extra costs of doing so.
Given that methanol can be ‘burnt’ in anything from a DMFC to conventional fuel cells to ICE engines to Stirling engines to gas turbines, there would seem to be quite a spread of extant manufacturers worldwide who could benefit from a diverse approach to bike-engine supply without starting from scratch, while the fact of diverse options would also help allow new entrants to get past incumbents’ obstructions.
In short, it seems that powered bikes/trikes/quads are the way that the pressures of ecology, economics and physics are pushing the society, and that we have a potentially very substantial supply of a sustainable hydrocarbon fuel if it is used with the efficiency that bikes would allow. Given the increasing unaffordability of car ownership in many nations, and the increasing urgency of ending fossil fuel combustion and of providing incentives for carbon sequestration globally, I’m hoping that you may choose to explore the maths of these possibilities in a future post.
Regards,
Lewis
Might as well recycle the CO2 from the exhaust of a fossil fueled plant… as direct air capture seems “impossible” at this time (unless a $600/tCO2 fee is imposed).
From a coal plant, it would be 12x less than air capture according to this in depth article…
http://www.aps.org/policy/reports/popa-reports/loader.cfm?csModule=security/getfile&PageID=244407
This allows for only one recycling (and much easier to deal with CO2 trading or taxing schemes). Process heat from a high temp nuclear reactor or from a concentrated solar setup will do the trick via hydrogen from thermo-splitting of water, also at high capital costs.
I can not seem to find out how much energy is required to make rechargeable batteries, but have a hunch that it is less energy and capital intensive.
Being neither a mathematician of Tom’s expertize nor a Nobel laureate chemist like George Olah, I’ve no useful comment on the latter’s proposal of collecting carbon from ambient air for methanol production, except to observe that the latter found the overall economics of doing so with hydropower as the energy source for the feedstocks’ production to be satisfactorily positive. Given that his proposal is of carbon capture for use in producing a valuable end product, as opposed to just lowering airborne CO2ppmv, the study you link is actually costing a rather different operation.
That said I’d be very interested to see Tom’s calculation of the comparative full-spectrum viability of the three potential methanol production sources posted above. With the scale of sea area with prevailing wave heights over 8ms, and with the WRI/WFN study showing around 1.6GHa.s globally available for afforestation without taking farm land, let alone Olah’s remote very large hydropower, the potential annual methanol output looks substantial. The core question is whether using that supply to power bikes actually gets the most valuable outcome for society in terms of mobility, or whether electric batteries for surface transport and methanol feedstock for say aviation fuel may offer a better outcome.
Regards,
Lewis
Hydroelectric is not the answer.
https://dothemath.ucsd.edu/2011/12/how-much-dam-energy-can-we-get/
Anybody promising a hydro-based solution is either peddling hopium and snake oil.
Nuclear fission is the only currently available primary energy source which can provide enough dependable capacity at a price which doesn’t destroy the economy in an energy trap(1). The cost of a nuclear kWh is currently high due to excessive bureaucracy and a lack of mass production hailing from an era before current passively safe designs.
There are only two realistic escape routes from the current situation:
1. Revolutionary innovation in reducing the cost of PV solar.
2. Next generation nuclear rolled out urgently as a matter of national security.
If we don’t see #1 or #2 then civilization as we know it is going down the toilet. Hydroelectric, windmills, biodiesel, gerbil wheels, etc. cannot maintain our current standard of living, which it itself a prerequisite for peace.
Comfortable, free people aren’t inclined to war, violence, or generally evil behavior. Sure, we can ration everything and move the masses into sustainable eco-ghettos, but that only replaces a resource problem with a social problem.
(1) https://dothemath.ucsd.edu/2011/10/the-energy-trap/
I should clarify that the word “cost” here refers to input costs, not a price net of subsidies. When renewable technologies are subsidized, someone must still pay the price, and if that price is too high, then we’re right back to the twin traps of energy and economics.
We can’t avert the impending economic collapse from peak oil unless we find a primary energy source offering a similar ratio of capital output to capital input.
But nuclear already is heavily subsidized by the government.
And all mainstream energy sources benefit from incomprehensibly massive subsidies in the form of free reign to pollute.
Coal is cheap for the plant operators, sure, but massively expensive for the rest of us. If the plant operators had to clean up after their mining operations and clean up the gigatons of pollution they pump into the atmosphere, coal-fired electricity would be just about the most expensive form of energy on the market.
We have cheap energy for the same reason that somebody with an outhouse in one corner of the bedroom has cheap indoor plumbing.
Once you add up all the true costs of energy production, solar is, far and away, the cheapest form of energy available. And it’s also so plentiful that we could power the entire global civilization just by covering all American suburban homes in solar panels.
Cheers,
b&
Right. And this someone, now-a-days, is effectively the fossil fuel consumer, because fossil fuels are negatively subsidized. They bring in royalties and excise tax income greatly exceeding the subsidies that are often mentioned.
True-ish. We in Ontario are looking at new nuclear construction, and the projected costs are on the order of $0.05 per kWh.
Nuclear fission is uniquely threatening to the fossil fuel income of the very bureaucracy you mention. “The flak is always heaviest over the target”.
AIUI solar PV’s been having an ongoing price revolution, and uptake in excess of projections.
New US nuclear power is tres expensive, but so is new 24/7 solar thermal. But Yglesias says the US has absurdly high infrastructure costs in general, compared to Japan or Europe, let alone China. China claims $1.50/watt nuclear but I’m scared about the safety of that.
I think this company in Germany is building quite an interesting solution to energy storage. They are claiming 54% efficiency in energy storage, which is much better than the number I put in the comment above (I am not sure if you approved it).
You might want to look at their web-site: http://www.solar-fuel.net
It sounds very exciting to me…
This was my idea, six years ago, for dealing with limited battery capacity. Works better for much smaller vehicles: http://dr2chase.wordpress.com/2006/10/10/so-when-we-run-out-of-oil-how-will-we-get-around/
As my 1st attempt to contribute to the conversation:
http://wheels.blogs.nytimes.com/2012/02/26/envia-claims-breakthrough-in-lithium-ion-battery-cost-and-energy-density/
$150/kwh is the game changer if true. Could the crossover be 18 months away?
I read these stories all the time, just not in the NYT.
Along with the electric bicycle, the velomobile also seems like a good candidate as cars keep getting far more out of reach for the common man. Perhaps the most efficient means of transport in history, the velomobile is also better than the bicycle for lugging stuff around and protecting against weather. The main drawback is going up hills, which is much easier with electric assist. Electrically assisted velomobiles are currently practically illegal in EU (since it’s very difficult to stay below the maximum speed), it’s a matter of unregistred vehicles, an easy problem to solve.
I have two electric assist bikes. I have been tinkering with them for years. It is impossible to find anyone to repair them. Bike shops are worried about liability; they don’t have the expertise to deal with electricity and potentially dangerous connections and batteries. It is not just that it is expensive to have someone work on them – it is that there is no one who can or will for the prices people are willing to pay. They are basically industrial products and face the same economic headwinds as all industrial products. There is no economic incentive to produce or maintain them because the people who would use them have no money.
This is all no doubt true, but it is a brain-dead situation. The electronics to run a bicycle are far from rocket science. The only thing really unusual about a bicycle (compared to a laptop or an automobile) is the vibration (I build my own bicycle lights, and vibration is THE problem).
Hi Tom,
I’d be interested, if you have time, to see you “do the math” on the two other large-scale energy storage prospects in the works, namely compressed air storage (using tanks, caverns, or giant submerged airbags) and chemical storage (usually beginning with electrolysis to hydrogen, and either storing as hydrogen or converting to methane, ammonia, or methanol). My understanding is that these technologies, especially chemical storage, scale better than pumped hydro and batteries but have significantly lower conversion efficiencies, thus requiring more wind turbines/solar panels (although this may not be a huge problem if the surplus intermittent energy would otherwise go to waste).
I’m an alternative energy researcher myself and have written some on our prospects for future energy (see linked blog post), and I would be willing to contribute “guest posts” on these topics or send you drafts that you could edit as you wish. I could use the excuse to do some more research in those areas myself.
I’ve always wondered how much energy it takes to make a battery, and finally found something.
From this, it seems that the ole PbA requires about 25 MJ/kg to make as compared to about 150 MJ/Kg for a li-ion…
http://www.transportation.anl.gov/pdfs/B/644.PDF
Hopefully, I am reading this wrong (table 2) as it seems it would take literally HUNDREDS of charges just to recoup EROEI !
Interesting and very useful study. They have a graph of cradle-to-gate energy in MJ/Wh, which can be easily converted to Wh(manufacturing energy)/Wh(storage capacity). Ignoring the error bars, these values are:
Lead-acid: 170 Wh/Wh
Li-ion: 470 Wh/Wh
Na-S or Ni-Cd: 500 Wh/Wh
NiMH: 750 Wh/Wh
Extrapolating on these figures, making a 16.5 kWh Chevy Volt Li-ion battery requires 7.76 MWh of energy inputs, which is equivalent to 212 gallons of gasoline.
My Volt saved me 520 gallons of gasoline in the first year compared to the Lexus (20 MPG) I was driving (45 gallons vs 565 gallons). Even compared to a 40 MPG car, it would save 237 gallons in one year. Over 8-10 years of usable life that’s 1,896 – 2,370 gallons saved.
Also, it is impossible for a battery to recoup EROEI, as it doesn’t produce any energy. While it’s true that after 470 cycles a lithium ion cell will have stored and release the amount of energy that was required to produce it, the EROEI of the entire system will depend on the energy produced (by wind, photovoltaic, coal, etc.) and the total energy invested (in production, storage, and use technologies).
Along these lines, many modern photovoltaic systems have embodied energy costs around 30 MJ/W (8300Wh/W), which works out to a 6-year energy payback time assuming four full-sun hours per day. (See http://gcep.stanford.edu/pdfs/ZEohxHyFp1f7TO2AP8mdwg/MichaelDale_GCEPposter2011.pdf Some newer technologies have much faster energy payback times, but I’m sticking with crystalline silicon as that is still the most common.)
Now let’s look at a hypothetical off-grid PV array with 2 kW of PV cells and 6 kWh of battery storage. The PV cells have embodied energy of 16.6 MWh. The batteries (assuming lead-acid at 170 Wh/Wh) have embodied energy of 1.02 MWh, but if average battery life is five years and we assume a 40-year system lifespan, then the batteries will need to be replaced eight times, for a total of 8.2 MWh. This is about half of the energy embodied in the panels, inverter, wires, etc., meaning that an off-grid system has a 50% longer energy payback time (around nine years) compared to a comparable grid-tied system.
Great job: thanks for the clear work. If you follow a “rule of thumb” for off-grid systems that the batteries should be sized for three days of zero input, and your average input is 4 hours per day, then you would want a battery bank of 24 kWh; four times bigger than the number you used. In this case, the batteries (including replacement) may triple the total energy invested over the PV system alone. Still net energy positive, but lower overall EROEI and energy payback.
Then again, when you size a system this large, deep cycles become rarer, and the battery replacement interval may increase significantly. I know someone in Maine with an off-grid system whose battery bank is 15 years old and doing fine. So realistically, we may well get back to your conclusion that the battery contribution is less than that of the PV system, in terms of energy.
This would again be subject to the same types of considerations as your previous article.
Specifically, if batteries are expensive in terms of manufacturing energy, it makes more sense to use a battery only big enough to make it through the night, and then to have enough panels to be sure that the battery is topped off even on a cloudy winter day.
If we ever come up with a really cheap battery (in terms of both energy to manufacture and cost to purchase), then it’d make sense to have enough battery capacity to go weeks (or even months) on end without input, and only enough panels for long-term net production.
Cheers,
b&
In an isolated small-scale system, having only one night’s battery storage means the input would need to handle the worst days, which can easily dip to 10% of a normal day several times a year. So this would require a 10× overbuild. Maybe this is fine, but at present it seems like too large a factor.
First, even assuming that the worst days only produce 10% of the average, you still don’t need a ten-fold overbuild. The battery only has to last overnight, not the full day. During the day, you’d draw power directly from the panels. I can’t do calculus in my head, but you’d need a five-fold overbuild to make it through the (roughly) twelve hours of night, minus your reduced energy needs when everybody is asleep, plus enough to provide power for daytime activities.
Next, you’re describing a worst-case scenario that I don’t think is typical. I’m at the other end of the scale, in Arizona. My overbuild is roughly 1.5, and it’s just about enough for an overnight battery to be all I’d need. It’s certainly enough for an all-day battery to do the trick…
…which leads to my last point. I presented the two extremes: overbuilt solar with an overnight battery, or an oversized battery with panels sized for the long-term average. The first works for dirt-cheap panels and solid-gold batteries; the second, for solid-gold panels and dirt-cheap batteries.
Reality is somewhere between, so the proper proportioning is somewhere between.
However, panels are generally cheaper than batteries and getting cheaper faster than batteries, so you’re generally going to be better off with more panels and a smaller battery.
That’s especially true when it comes to your thought experiment of a nation-sized battery. You made it clear that there’s no way we’ll ever have enough battery material for a week’s worth of storage. However, elsewhere, you’ve made the point that just covering all suburban carports would be enough to meet our annual energy needs. Expand that to all suburban roofs, and there’s enough overbuild to only need an overnight national battery. Add in a nationwide smart grid so excesses in sunny states can power deficits in stormy ones, and our national battery shrinks to something like 1/20 the size of your week-long battery, and it again enters the realm of feasibility.
Of course, if storage were cheap and production and deployment of panels were expensive, I’d be observing how we could get by with only covering carports so long as we built your nation-sized week-long battery, but that’s the opposite of the world we find ourselves in.
Cheers,
b&
Good points; I’ll certainly buy that we often find ourselves between the extreme situations!
But I’ll stand by the 10× overbuild if one needs to deal with a 10% day. Imagine you typically use 20 kWh in a day. The batteries need to provide 10 kWh overnight (keeping things simple and splitting right in half). If the average day delivers 20 kWh to your PV array, and a day rolls along providing 2 kWh instead, you won’t have enough energy to satisfy your night or day demand. You would need a 10× larger PV array to proved 20 kWh total: 10 of which you would use during the day, and 10 at night.
That said, I mistook the factor of ten I see as relative to yearly average, when in fact it’s relative to the biggest days: so my range spans a factor of ten. The annual average is about 63% of the peak days. So my worst days are about 15% of the annual average, not 10%. And this is San Diego. The really dim days are the days with lots of rain. And San Diego doesn’t have many of these days (10 inches (25 cm) per year). So I imagine many “normal” places deal with 10–15% days several times per year, FWIW.
Ah — thanks. I see your point about the overbuild factor.
The last piece of the puzzle would be the demand for energy at times of minimal production.
Here in Arizona, wintertime energy consumption is well below the annual average, which is why I with my modest overbuild wouldn’t need much in the way of battery backup. Indeed, the battery I’d need to keep the airconditioner going through the middle of an August night would be more than ample to make it through a winter storm.
If your peak consumption is associated with wintertime heating, you may need a big overbuild and big battery both…or a wire (grid) that stretches all the way to places like Arizona….
Cheers,
b&
I used the term EROEI for lack of a better explanation. Anyways, thanks for clarifying.
The Volt and other electric and hybrid cars currently available on the market are far too big for efficient utilization of the technology.
I would believe a feasible size for a vehicle dedicate to private transportation using electric engines would be similar in size of smart cars. Far less energy would be required to operate such a sized vehicle.
If everyone was driving a much smaller/lighter car at speeds below 30 MPH all the time I’d agree. But over here in the real world, with 5,000 lb SUV’s going 80 MPH, I feel safer in my Volt. 🙂 Look at the article on the 100 MPG car on this site, weight is a secondary factor, not very important at all actually.
The problem is not only one of range. Its also one of irrational expectations. If we would just slow down, energy requirements would drop precipitously see here.
http://ecomodder.com/forum/tool-aero-rolling-resistance.php at bicycle/horse speeds solar powered cars become viable.
If you slow down then you can’t go as far, and you don’t have as much space in which to park all those cars.
Good article Tom. I have solar, an all electric Leaf and lead acid to back up my solar, as well.
I have the all electric Leaf because I run it on Solar and I offset my solar costs against gasoline. Put that in your calculations. Moreover, I anticipate the high likelihood that gasoline will explode in cost, (watching the supply demand curve and declining export availability along with our 95%+ exposure to liquid fuels in order to move anything), if it is readily a available at any price for all of us at some soon point. Then, how will our economy move?
I know you have thought about that and it seems to me that a sudden oil shock, one our colleague Chris Martenson is anticipating this next year, is in the cards. And we do not control our own deck of cards. Doubling of oil price will shut down the economy here in the southwest. We are totally dependent on cars and trucks for all economic activity.
I suggest that all electric transportation (even electric bikes, trikes and small motorcycles) run on solar are a prudent and wise investment for those of us in the “peak oil world.”
I don’t yet make enough money to convert over, to solar powered electric bikes (I’ll need five of ’em). But can’t wait to be able to do so.
However, if the financial crash comes (for any reason), I believe it won’t do any good, anyways.
A previous poster found posts to envia systems. I found the following press release : http://enviasystems.com/announcement/
Note that the “full report is available on request”. Perhaps you, Tmurphy, should click that link and ask these guys to send you the full paper written by scientists working for the “Naval Surface Warfare Center”.
It looks pretty darn convincing. IF what they are doing actually has these performance numbers, that’s half the problem right there. The other problem is cost : you could probably get an estimate of “true, long term manufacturing cost” by multiplying the cost of the raw materials they claim to use by 5 or something.
They claim half the cost of current packs, but again, until they actually have a catalog with these cells listed that’s just a marketing number.
If all this works, your whole post is obsolete. Maybe in 2013 this problem will be officially solved.
I think we have to be careful comparing the volumetric energy density of batteries vs. fuel. In reality we should also include the volume of the whole ICE and exhaust system for a regular car. Electric motors are tiny in comparison. The Tesla Model S has trunk space in the front and rear because there is no ICE and the batteries are in the floor.
Regarding the energy needed to make such a transition happen, we may still be decades away from Peak Coal (it has been pointed out to me that the world’s estimates for recoverable coal are based on today’s prices only, and we may ultimately have quite a bit more at high prices). The question is: if we do have an extra few decades because of coal, do we use that as an opportunity to develop a renewable infrastructure or instead to fuel further economic growth taking us deeper into ecological overshoot?
Also, I have to question why everyone expects a new electric transportation infrastructure to be as or more convenient than what we have today. Certainly to be competitive today it does, but in the future I think, “Is it convenient?” will take a back seat to “Is it possible?” Even today’s EV’s meet that challenge for 99% of the uses out there, my Leaf does with minor lifestyle adjustments. For the simple reason that people will work less in the future we won’t have to race all over the place with lightning speed like we do now.
I have just emerged from a week in the wilderness photographing grizzlies, wolves, and humpbacks with my 4 big DSLR’s, 4 video cameras, and lots of other electronic stuff. All are chargeable with my marine grade solar panel. All except for my computer which is being plugged in right now, powered with diesel presumably. I am at the Hakai Beach Institute station on BC’s central coast and I noticed they have installed some big solar panels in the back.
I’ve been away from power for a week and could get by with all my electronics for a month without power if I needed to, I can get it all done, this idea of effortless convenience is a concept that I think only has relevance in today’s high paced world of exponential economic growth. Anyways, back into the wilderness for another week…
Note: the author of the APS article referenced in the post wrote an extended version that is worth a look…some more useful details.
Hi Tom,
Unfortunately, I agree with your conclusion that batteries are not the panacea we are lead to believe. Batteries storage densities (140 kJ/kg for lead/acid or 360 kj/kg for Li) are about 1/1000 of the storage density of gasoline (36 MJ/kg) – I prefer using timeless units to avoid confusion.
Furthermore, as you rightly point out, there are physical limits which curtail possible improvements and therefore, highly unlikely to “solve” the transportation problem.
Politicians at the US DoE overrule technocrats and publish goals for the requirements for EV, naively believing that by stating them, the scientific community, entrepreneurs and VCs are going to come with the product tomorrow or next day. You, me and others, can point out faults. The attempts provided so far, are not solutions, but compromises, hoping to generate some cash flow to continue researching.
However, I am faced with a dilemma. It is easy to say – this does not work, this other does not either, this one is expensive, this other is complicated. We become “critics” same as movie, books or restaurant critics. It is easy to criticize. I do not want to be a critic. I want to offer constructive solutions.
As some other has suggested, it can solve a portion of the transportation problem, if we give up our demands of hauling two tons of metal around us, for a trip to the grocery store. It is not rocket science, but the problem is similar to rockets. You need fuel to lift the fuel.
There is no thermodynamic requirement to haul 2+ tons to the grocery store. Given the state of the art of current batteries, we might be able to have a 500 kg vehicle capable of driving the stated goal of 300 miles without recharging. With less weight, the vehicle might costs ¼ of the Volt so that you can buy one and have some extra cash. Many efforts are going that direction – electric bicycles, motorcycles and three wheelers.
If I cannot contribute with positive, constructive, realistic solutions, I am as bad as uninformed deniers suggesting there is plenty of oil and climate change is a natural phenomenon. I am aware that there is no magic solution. I will attempt to continue pointing out the best alternatives.
Cheers,
eduardo
Great article, as usual. However, it is perhaps relevant to add that presently at least one (admittedly, highly expensive) electric vehicle is getting close to the 300 miles per charge goal (Tesla’s Model S, using an 85kWh battery) – and other vehicles will surely follow.
The question is this: your article seems to put beyond reach the substitution of ICE vehicles with electric vehicles from a technological standpoint; but even taking into account the difference in specific energy between Li-Ion batteries and gasoline, how unlikely is it that battery prices will drop enough (say, by 75% in the next 10 years) to make the additional battery weight affordable, and thus electric vehicles commercially competitive with ICE vehicles?
How about a “smart” car that is powered for about 150 miles on the much safer (and swapable?) lifepo4 battery. This link seems to convince me that we DO have enough lithium to power many billions of such little cars.
http://gas2.org/2008/10/13/lithium-counterpoint-no-shortage-for-electric-cars/
Better than a bike in bad weather, and good enough for me because I would rather deal with the “limited” mileage, than having to pay 50 cents per mile as I do now!
The current vehicle size problem is directly tied to the population problem.
Anything which isn’t a “family” vehicle will have trouble with mass market penetration. Tiny econoboxes and sports cars might sell, but only in niche groups who aren’t relying on them for family transportation.
You can’t transport a whole family and the occasional friend in one of the proposed radically different efficiency machines. Enclosed bicycles? Compact two seaters? Forget about it.
Even an exceptionally small and efficient four seat coffin on wheels wouldn’t sell enough units to make a sizeable impact because it wouldn’t be safe enough for the children (won’t somebody please think of the children?).
Two kids, plus kid paraphrenalia, family groceries, etc. will never be compatible with the tiny “reimagined” vehicles. The problems are magnified substantially with three or more in tow. Now add in all the extracurricular activities, doctors visits, and mom’s need to haul all of them around as a sitter and the small cars become a non-starter for the mainstream.
The size and safety concerns preclude any size/weight reduction for mainstream use. The mainstream lifestyle dictates the current form factor.
Once again, we’re back to that pesky population problem which underlies every resource issue, yet is so uncomfortable for most people to discuss.
Sorry about using the “smart” car as an example (I should know better).
However, in light of future challenges, people may have to re-adjust to using smaller cars. The only difference is lightweight materials and like a 150 mile range. The kids will fit just fine unless it’s a “smart” car. Speaking of such, I used to have a 3 cylinder Geo which drove 5 people around on about the same gas mileage as the “smart” car (so, being decades later, it isn’t smart at all, just inappropriately much smaller).
Therefore, an electric Geo sized car (or slightly larger to account for LiFePO4 batteries) should satisfy all but the requirements of the ego.
The safety is partly a matter of what else is on the road. One might ‘need’ an SUV to protect yourself from other SUVs, but there’s a legislative fix for that coordination problem.
Not all the stuff you describe is a population problem; some of it’s an expectations problem. The “extracurricular activities”, for example; kids used to play outside with the neighbors, rather than having their lives planned. Ditto for “mom’s need to haul them around.” Older kids can take transit to whatever they’re doing, if their is transit
Hi Tom,
This is a terrific and necessary discussion.
I want to point out that In a full cost analysis comparing electric to internal combustion engines, you have omitted certain necessary inputs and their costs, in particular to an internal combustion engine running on gasoline, for example lubricant (motor oil) which over 62,000 miles is quite a few quarts and this probably narrows the cost differential somewhat. I am less familiar with maintenance costs of electric motors, but I don’t see how we can arrive at a true comparison without taking into account all the entropic factors.
Just found this…. US automaker General Motors Co. has announced it will halt production of the Chevrolet Volt electric car for four weeks, citing the car’s failure to meet targeted sales projections, according to Bloomberg news.
GM sold 10,666 Volts in the US in July. Global sales were targeted at 60,000 units, with 45,000 on the US market. Sales for 2011 were also under target, and even though an investigation into vehicle safety concluded that the Volt did not pose a fire risk, the congressional hearings on the issue led to a slow-down in sales.
http://oilprice.com/Latest-Energy-News/World-News/GM-Temporarily-Halts-Volt-Production-over-Low-Sales.html
So, what if the Volt is a flop. Will you be able to buy a new battery bank for it in ten years time…….?
The thing that’s left out of this (and I’m not being critical, merely observational) is economic collapse. All the stuff discussed on this thread relies on a vulnerable complex system, which in my opinion can only fail.
Makes for an interesting new unit: GM sold 10.7 kilovolts last month…
Bit of nerd humor: folks who are amped on the Volt make powerful advocates.